Federal Agency for Education
Saratov State Socio-Economic University
Marks branch
Department of General Humanitarian Disciplines
ESSAY
According to "Physics"
on the topic: “Electric current. Ohm's law"
2nd year student
Specialty: "Eup pp"
Checked by: Starikova N.N.
Marx - 2010
Electricity. Ohm's law
If an insulated conductor is placed in electric field
then a force will act on the free charges q in the conductor. As a result, a short-term movement of free charges occurs in the conductor. This process will end when the own electric field of the charges that have arisen on the surface of the conductor completely compensates for the external field. The resulting electrostatic field inside the conductor will be zero (see § 1.5).However, in conductors, under certain conditions, a continuous ordered movement of free electric charge carriers can occur. This movement is called electric current. The direction of movement of positive free charges is taken as the direction of the electric current. For the existence of an electric current in a conductor, it is necessary to create an electric field in it.
A quantitative measure of the electric current is the strength of the current I - scalar physical quantity, equal to the ratio of the charge Δq transferred through the cross section of the conductor (Fig. 1.8.1) over the time interval Δt, to this time interval:
If the strength of the current and its direction do not change with time, then such a current is called constant.
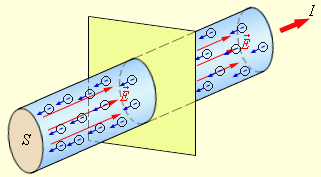
Figure 1.8.1.
The ordered movement of electrons in a metal conductor and the current I. S is the cross-sectional area of the conductor,
- electric fieldIn the International System of Units SI, current is measured in amperes (A). The current unit 1 A is set according to magnetic interaction two parallel conductors with current (see § 1.16).
Constant electricity can only be created in a closed circuit in which free charge carriers circulate along closed paths. The electric field at different points in such a circuit is constant over time. Therefore, the electric field in the circuit direct current has the character of a frozen electrostatic field. But when moving an electric charge in an electrostatic field along a closed path, the work of electric forces is zero (see § 1.4). Therefore, for the existence of direct current, it is necessary to have in electrical circuit a device capable of creating and maintaining potential differences in circuit sections due to the work of forces of non-electrostatic origin. Such devices are called direct current sources. Forces of non-electrostatic origin acting on free charge carriers from current sources are called external forces.
The nature of outside forces can be different. In galvanic cells or batteries, they arise as a result of electrochemical processes; in DC generators, external forces arise when conductors move in a magnetic field. The current source in the electrical circuit plays the same role as the pump, which is necessary for pumping fluid in a closed hydraulic system. Under the action of external forces, electric charges move inside the current source against the forces of the electrostatic field, due to which a constant electric current can be maintained in a closed circuit.
When electric charges move along a DC circuit, external forces acting inside current sources do work.
A physical quantity equal to the ratio of the work A st of external forces when the charge q moves from the negative pole of the current source to the positive to the value of this charge is called the electromotive force of the source (EMF):
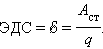
Thus, the EMF is determined by the work done by external forces when moving a single positive charge. The electromotive force, like the potential difference, is measured in volts (V).
When a single positive charge moves along a closed DC circuit, the work of external forces is equal to the sum of the EMF acting in this circuit, and the work of the electrostatic field is zero.
The DC circuit can be divided into separate sections. Those sections on which external forces do not act (i.e., sections that do not contain current sources) are called homogeneous. Sections that include current sources are called heterogeneous.
When a unit positive charge moves along a certain section of the circuit, both electrostatic (Coulomb) and external forces do work. The work of electrostatic forces is equal to the potential difference Δφ 12 \u003d φ 1 - φ 2 between the initial (1) and final (2) points of the inhomogeneous section. The work of external forces is by definition electromotive force
12 operating in this area. So the total work isU 12 \u003d φ 1 - φ 2 +
12 .The value of U 12 is usually called the voltage in the circuit section 1–2. In the case of a homogeneous section, the voltage is equal to the potential difference:
U 12 \u003d φ 1 - φ 2.
The German physicist G. Ohm in 1826 experimentally established that the strength of the current I flowing through a homogeneous metal conductor (i.e., a conductor in which no external forces act) is proportional to the voltage U at the ends of the conductor:
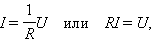
where R = const.
The value of R is usually called electrical resistance. A conductor with electrical resistance is called a resistor. This ratio expresses Ohm's law for a homogeneous section of the circuit: the current strength in the conductor is directly proportional to the applied voltage and inversely proportional to the resistance of the conductor.
In SI, the unit of electrical resistance of conductors is the ohm (Ohm). A resistance of 1 ohm has a section of the circuit in which, at a voltage of 1 V, a current of 1 A occurs.
Conductors obeying Ohm's law are called linear. The graphical dependence of the current strength I on the voltage U (such graphs are called current-voltage characteristics, abbreviated as CVC) is depicted by a straight line passing through the origin. It should be noted that there are many materials and devices that do not obey Ohm's law, for example, semiconductor diode or gas lamp. Even metal conductors at currents of sufficiently large strength, a deviation from Ohm's linear law is observed, since electrical resistance metal conductors increases with increasing temperature.
For a circuit section containing EMF, Ohm's law is written in the following form:
IR \u003d U 12 \u003d φ 1 - φ 2 +
= Δφ 12 + .This relation is usually called the generalized Ohm's law or Ohm's law for an inhomogeneous chain section.
On fig. 1.8.2 shows a closed DC circuit. The chain section (cd) is homogeneous.
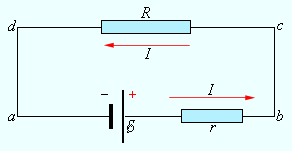
Figure 1.8.2.
DC circuit
Ohm's law
Section (ab) contains a current source with an EMF equal to
.According to Ohm's law for a heterogeneous area,
.Adding both equalities, we get:
I (R + r) = Δφ cd + Δφ ab +
.But Δφ cd = Δφ ba = – Δφ ab. That's why
This formula will express Ohm's law for complete chain: the current strength in a complete circuit is equal to the electromotive force of the source, divided by the sum of the resistances of the homogeneous and inhomogeneous sections of the circuit.
The resistance r of the inhomogeneous section in fig. 1.8.2 can be seen as internal resistance current source. In this case, section (ab) in Fig. 1.8.2 is the internal section of the source. If points a and b are closed with a conductor whose resistance is small compared to the internal resistance of the source (R<< r), тогда в цепи потечет ток короткого замыкания
Short circuit current - the maximum current that can be obtained from a given source with an electromotive force
and internal resistance r. For sources with low internal resistance, the short-circuit current can be very large and cause the destruction of the electrical circuit or source. For example, lead-acid batteries used in automobiles can have a short circuit current of several hundred amperes. Particularly dangerous are short circuits in lighting networks powered by substations (thousands of amperes). To avoid the destructive effect of such high currents, fuses or special circuit breakers are included in the circuit.In some cases, to prevent dangerous values of the short circuit current, some external resistance is connected in series to the source. Then the resistance r is equal to the sum of the internal resistance of the source and the external resistance, and in the event of a short circuit, the current strength will not be excessively large.
If the external circuit is open, then Δφ ba = – Δφ ab =
, i.e., the potential difference at the poles of an open battery is equal to its EMF.If the external load resistance R is turned on and current I flows through the battery, the potential difference at its poles becomes equal to
– Ir.On fig. 1.8.3 is a schematic representation of a DC source with an EMF equal to
and internal resistance r in three modes: “idling”, work on load and short circuit mode (short circuit). The strength of the electric field inside the battery and the forces acting on positive charges are indicated: – electric force and – third-party force. In short circuit mode, the electric field inside the battery disappears.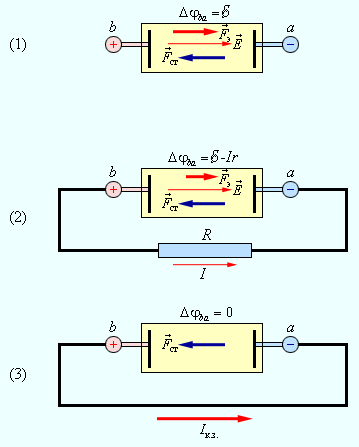
Figure 1.8.3.
Schematic representation of a DC source: 1 - the battery is open; 2 - the battery is closed to external resistance R; 3 - short circuit mode
To measure voltages and currents in DC electrical circuits, special devices are used - voltmeters and ammeters.
The voltmeter is designed to measure the potential difference applied to its terminals. It is connected in parallel with the section of the circuit on which the potential difference is measured. Any voltmeter has some internal resistance R B . In order for the voltmeter not to introduce a noticeable redistribution of currents when connected to the measured circuit, its internal resistance must be large compared to the resistance of the section of the circuit to which it is connected. For the circuit shown in Fig. 1.8.4, this condition is written as:
R B >> R 1 .
This condition means that the current I B \u003d Δφ cd / R B flowing through the voltmeter is much less than the current I \u003d Δφ cd / R 1 that flows through the tested section of the circuit.
Since there are no outside forces acting inside the voltmeter, the potential difference at its terminals coincides, by definition, with the voltage. Therefore, we can say that the voltmeter measures voltage.
The ammeter is designed to measure the current strength in the circuit. The ammeter is connected in series to the break in the electrical circuit so that the entire measured current passes through it. The ammeter also has some internal resistance R A . Unlike a voltmeter, the internal resistance of an ammeter must be sufficiently small compared to the total resistance of the entire circuit. For the circuit in fig. 1.8.4 the resistance of the ammeter must satisfy the condition
R A<< (r + R 1 + R 2),
so that when the ammeter is turned on, the current in the circuit does not change.
Measuring instruments - voltmeters and ammeters - are of two types: pointer (analog) and digital. Digital electrical meters are complex electronic devices. Usually digital instruments provide higher measurement accuracy.
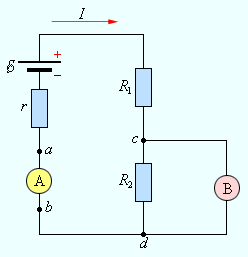
Figure 1.8.4.
Inclusion of an ammeter (A) and a voltmeter (B) in an electrical circuit
Series and parallel connection of conductors
Conductors in electrical circuits can be connected in series and in parallel.
With a series connection of conductors (Fig. 1.9.1), the current strength in all conductors is the same:
I 1 \u003d I 2 \u003d I.
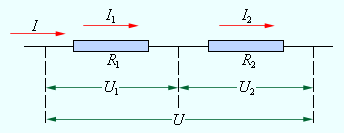
Figure 1.9.1.
Serial connection of conductors
According to Ohm's law, the voltages U 1 and U 2 on the conductors are equal
U 1 = IR 1, U 2 = IR 2.
The total voltage U on both conductors is equal to the sum of the voltages U 1 and U 2:
U \u003d U 1 + U 2 \u003d I (R 1 + R 2) \u003d IR,
where R is the electrical resistance of the entire circuit. This implies:
R \u003d R 1 + R 2.
When connected in series, the total resistance of the circuit is equal to the sum of the resistances of the individual conductors.
This result is valid for any number of series-connected conductors.
With a parallel connection (Fig. 1.9.2), the voltages U 1 and U 2 on both conductors are the same:
U 1 \u003d U 2 \u003d U.
The sum of currents I 1 + I 2 flowing through both conductors is equal to the current in an unbranched circuit:
I \u003d I 1 + I 2.
This result follows from the fact that no charges can accumulate at the branching points of the currents (nodes A and B) in a DC circuit. For example, charge IΔt flows to node A in time Δt, and charge I 1 Δt + I 2 Δt flows away from node A in the same time. Therefore, I = I 1 + I 2 .
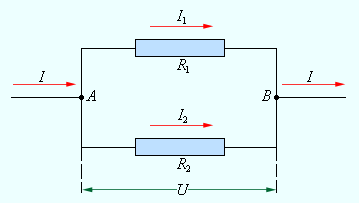
Figure 1.9.2.
Parallel connection of conductors
Writing based on Ohm's law
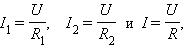
where R is the electrical resistance of the entire circuit, we get
When the conductors are connected in parallel, the reciprocal of the total resistance of the circuit is equal to the sum of the reciprocals of the resistances of the parallel-connected conductors.
This result is valid for any number of conductors connected in parallel.
Formulas for series and parallel connection of conductors allow in many cases to calculate the resistance of a complex circuit consisting of many resistors. On fig. 1.9.3 gives an example of such a complex circuit and indicates the sequence of calculations.
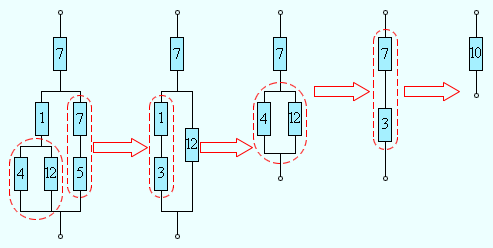
Figure 1.9.3.
Calculation of the resistance of a complex circuit. All conductor resistances are in ohms (Ohm)
It should be noted that not all complex circuits consisting of conductors with different resistances can be calculated using formulas for series and parallel connection. On fig. 1.9.4 shows an example of an electrical circuit that cannot be calculated using the above method.
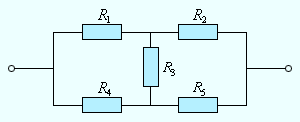
Figure 1.9.4.
An example of an electrical circuit that is not reducible to a combination of series and parallel conductors
DC electric current
Conditions for the occurrence of current.
An electric current is a directed movement of charged particles. The quantitative characteristics of the current are its current strength (the ratio of the charge: transferred through the cross section of the conductor per unit time):
and its density, determined by the ratio:
The unit of current strength is the ampere (1A is the characteristic value of the current consumed by household electric heaters).
The necessary conditions for the existence of current are the presence of free charge carriers, a closed circuit and an EMF source (battery) that supports directional movement.
Electric current can exist in various media: in metals, vacuum, gases, solutions and melts of electrolytes, plasma, semiconductors, tissues of living organisms.
When a current flows, the interaction of charge carriers with the environment almost always occurs, accompanied by the transfer of energy to the latter in the form of heat. The role of the EMF source is precisely to compensate for heat losses in the circuits.
Electric current in metals is due to the movement of relatively free electrons through the crystal lattice. The reasons for the existence of free electrons in conducting crystals can only be explained in the language of quantum mechanics.
Experience shows that the strength of the electric current flowing through the conductor is proportional to the potential difference applied to its ends (Ohm's law). The coefficient of proportionality between current and voltage, which is constant for the selected conductor, is called electrical resistance:
Resistance is measured in ohms (the resistance of the human body is about 1000 ohms). The magnitude of the electrical resistance of conductors slightly increases with increasing temperature. This is due to the fact that when heated, the nodes of the crystal lattice increase chaotic thermal vibrations, which prevents the directed movement of electrons. In many problems, direct allowance for lattice vibrations turns out to be very laborious. To simplify the interaction of electrons with oscillating nodes, it turns out to be convenient to replace them with collisions with gas particles of hypothetical particles - phonons, whose properties are chosen so as to obtain a description as close as possible to reality and can turn out to be very exotic. Objects of this type are very popular in physics and are called quasiparticles. In addition to interactions with vibrations of the crystal lattice, the movement of electrons in a crystal can be hindered by dislocations - violations of the regularity of the lattice. Interactions with dislocations play a decisive role at low temperatures, when thermal vibrations are practically absent.
Some materials at low temperatures completely lose their electrical resistance, passing into a superconducting state. Current in such media can exist without any EMF, since there are no energy losses in collisions of electrons with phonons and dislocations. The creation of materials that retain the superconducting state at relatively high (room) temperatures and low currents is a very important task, the solution of which would make a real revolution in modern energy, because. would allow the transmission of electricity over long distances without heat loss.
Currently, electric current in metals is mainly used to convert electrical energy into heat (heaters, light sources) or mechanical (electric motors). In the latter case, the electric current is used as a source of magnetic fields, the interaction with which other currents cause the appearance of forces.
Electric current in vacuum is strictly speaking impossible due to the absence of free electric charges in it. However, some conductive substances, when heated or irradiated with light, are capable of emitting electrons from their surface (thermal emission and photoemission), which are able to maintain an electric current, moving from the cathode to another (positive) electrode - the anode. When a negative voltage is applied to the anode, the current in the circuit breaks. The described property causes the widespread use of electrovacuum devices in electronic devices for rectifying alternating current. Until relatively recently, electrovacuum devices were widely used as amplifiers of electrical signals. At present, they are almost completely replaced by semiconductor devices.
At first glance, electric current in gases cannot exist due to the absence of free charged particles (electrons in atoms and molecules of gases are firmly “linked” to nuclei by electrostatic forces). However, when transferring an energy of the order of 10 eV to an atom (the energy acquired by a free electron when passing through a potential difference of 10 V), the latter passes into an ionized state (an electron leaves the nucleus for an arbitrarily large distance). In gases at room temperature, there is always a very small amount of ionized atoms that have arisen under the action of cosmic radiation (photoionization). When such a gas is placed in an electric field, charged particles begin to accelerate, transferring the accumulated kinetic energy to neutral atoms and ionizing them. As a result, an avalanche-like process of increasing the number of free electrons and ions develops - an electric discharge occurs. The characteristic glow of the discharge is associated with the release of energy during the recombination of electrons and positive ions. The types of electrical discharges are very diverse and strongly depend on the composition of the gas and external conditions.
Plasma.
A substance containing a mixture of neutral atoms, free electrons and positive ions is called plasma. The plasma resulting from relatively low-current electrical discharges (for example, in “daylight” tubes) is characterized by very low concentrations of charged particles compared to neutral ones (). Usually it is called low-temperature, since the temperature of atoms and ions is close to room temperature. The average energy of much lighter electrons turns out to be much higher. That. low-temperature plasma is essentially a non-equilibrium, open medium. As noted, self-organization processes are possible in such media. A well-known example is the generation of highly ordered coherent radiation in the plasma of gas lasers.
The plasma can also be in thermodynamic equilibrium. For its existence, a very high temperature is required (at which the energy of thermal motion is comparable to the ionization energy). Such temperatures exist on the surface of the Sun, can occur during very powerful electrical discharges (lightning), during nuclear explosions. Such a plasma is called hot.
atmospheric electricity.
Earth is a fairly good conductor of electricity (compared to dry air). At an altitude of about 50 km, ionizing cosmic radiation causes the presence of the ionosphere - a layer of highly ionized gas. Measurements show that there is a huge potential difference between the ionosphere and the Earth's surface (about 5,000,000 V), and the ionosphere has a positive charge relative to the Earth. The presence of a potential difference between the Earth and the “sky” leads to the appearance of a current of very low density (A /) even in such a poor conductor as air. The total current coming to the surface of the planet is very large (approx. A), and the power it releases is comparable to the power of all built power plants (W). Natural questions arise about the mechanism for maintaining this potential difference and about the reasons why its presence has not yet been used in any way by man.
It has now been established that the main mechanism that charges the “sky” relative to the Earth is thunderstorms. Drops of water and ice crystals, moving down to the base of the thundercloud, collect negative charges in the atmosphere and thereby charge the lower part of the thundercloud with negative electricity to potentials many times greater than the potential of the Earth. As a result, a very large electric field arises between the Earth and the cloud, directed in the opposite direction compared to the field that exists in cloudless weather. Near conducting objects protruding from the Earth's surface, this field is further enhanced and is sufficient for gas ionization, which grows according to an avalanche law. The result is a very powerful electrical discharge called lightning. Contrary to popular belief, lightning starts on Earth and hits clouds, not the other way around.
An electric field of 100 V/m, which is characteristic of clear weather, cannot be used or even felt, although at a height equal to the height of a person, in its absence, it creates a potential difference of about 200 V. The reason for this is the low conductivity of the air and, as a result, the low densities of the currents flowing to the Earth's surface. The introduction of a good conductor (a person) into the electrical circuit, shunting a two-meter air column, practically does not change the total resistance of the “heaven-Earth” circuit, the current in which remains unchanged. The voltage drop caused by it on the human body is about U = IR = 0.2 μV, which lies significantly below the sensitivity threshold of our body.
Electric current in living tissues.
The important role of electrical impulses for the life of organisms was assumed more than 200 years ago. It is now known that these impulses are used to ensure the control of the work of organs and the transfer of information between them in the process of life. The role of cables for signal transmission in the most complex "biological computer" is played by nerves, which are based on highly specialized cells - neurons. The main functions of these cells are receiving, processing and amplifying electrical signals. Neurons communicate with each other in a “network” with the help of special elongated outgrowths - axons that act as conductors. Studies of the propagation of electrical signals in axons were carried out jointly by biologists, chemists and physicists in the 30-60s of our century and were one of the first successful examples of fruitful cooperation between representatives of related natural sciences.
As it turned out, the properties of electrical impulses propagating in axons differ significantly from those familiar to electrical engineering: 1) the speed of propagation of impulses along the axon turns out to be several orders of magnitude less than those characteristic of metal ones; 2) after the passage of an electrical impulse, there is a “dead” time during which the propagation of the next impulse is impossible; 3) there is a threshold voltage value (pulses with an amplitude below the threshold do not propagate); 4) with a slow increase in voltage, even to a value exceeding the threshold, the impulse is not transmitted along the axon (“accommodation”).
The listed peculiarities of axon conductivity, which are uncharacteristic for traditional electrical engineering, were explained within the framework of a very specific electrochemical mechanism, the central role in which belongs to a semi-permeable cell membrane for ions, which separates the internal volume of the cell (and its axon) containing an abnormally high concentration of K + ions and low - Na + from an environment filled with saline. As a result of the chaotic thermal motion of particles across the boundary between areas with different concentrations of positive ions, diffusion flows arise (K + - from the cell, Na + - inside it), the speeds of which are regulated by the permeability of the cell membrane and the electrical potential difference on both sides of it. Changes in the permeability of the membrane for each of the ions leads to a change in the number of charged particles crossing the boundary and, consequently, to a change in the electric potential of the axon relative to the external environment. Experiments have shown that the conductivity of a section of the membrane varies depending on the potential difference applied to it. That. the electrical impulse applied to the axon section changes the membrane conductivity for a short time (depending on the properties of the axon), which leads to a redistribution of charges, amplification of the impulse and the formation of its trailing edge. In this case, the axon simultaneously plays the role of a conductor and "amplifying substations - repeaters", which makes it possible to avoid attenuation of signals transmitted in the body over sufficiently long distances.
It is interesting that a very similar problem with the one that was solved by nature, shortly before the discovery of the mechanism of axon conduction, had to be solved in radio engineering when trying to organize a trans-Atlantic cable connection. In order to avoid attenuation and signal distortion in a long line, the cable had to be divided into relatively short links, between which amplifiers were placed. The experience gained by physicists in the creation of long cable communication lines has greatly facilitated the solution of the problem of the mechanism of axon electrical conductivity.
Bibliography
For the preparation of this work, materials from the site were used.
For the existence of a direct electric current, the presence of free charged particles and the presence of a current source are necessary. in which the conversion of any type of energy into the energy of an electric field is carried out.
Current source - a device in which any type of energy is converted into the energy of an electric field. In a current source, external forces act on charged particles in a closed circuit. The reasons for the appearance of external forces in various current sources are different. For example, in batteries and galvanic cells, external forces arise due to the flow of chemical reactions, in generators of power plants they arise when a conductor moves in a magnetic field, in photocells - when light acts on electrons in metals and semiconductors.
The electromotive force of the current source called the ratio of the work of external forces to the value of the positive charge transferred from the negative pole of the current source to the positive.
Basic concepts.
Current strength - a scalar physical quantity equal to the ratio of the charge that has passed through the conductor to the time for which this charge has passed.
where I - current strength, q - amount of charge (amount of electricity), t - charge transit time.
current density - vector physical quantity equal to the ratio of the current strength to the cross-sectional area of the conductor.
where j -current density, S - cross-sectional area of the conductor.
The direction of the current density vector coincides with the direction of motion of positively charged particles.
Voltage - scalar physical quantity equal to the ratio of the total work of the Coulomb and external forces when moving a positive charge in the area to the value of this charge.
where A - full work of third-party and Coulomb forces, q - electric charge.
Electrical resistance - a physical quantity characterizing the electrical properties of a circuit section.
where ρ - specific resistance of the conductor, l - the length of the conductor section, S - cross-sectional area of the conductor.
Conductivity is the reciprocal of the resistance
where G - conductivity.
Ohm's laws.
Ohm's law for a homogeneous section of a chain.
The current strength in a homogeneous section of the circuit is directly proportional to the voltage at a constant section resistance and inversely proportional to the section resistance at a constant voltage.
where U - tension in the area R - section resistance.
Ohm's law for an arbitrary section of the circuit containing a direct current source.
where φ 1 - φ 2 + ε = U voltage in a given section of the circuit,R - electrical resistance of a given section of the circuit.
Ohm's law for a complete circuit.
The current strength in a complete circuit is equal to the ratio of the electromotive force of the source to the sum of the resistances of the external and internal sections of the circuit.
where R - electrical resistance of the outer section of the circuit, r - electrical resistance of the internal section of the circuit.
Short circuit.
It follows from Ohm's law for a complete circuit that the current strength in a circuit with a given current source depends only on the resistance of the external circuit R.
If a conductor with resistance is connected to the poles of the current source R<< r, then only the EMF of the current source and its resistance will determine the value of the current in the circuit. This value of the current strength will be the limit for this current source and is called the short circuit current.
Electromotive force. Any current source is characterized by electromotive force, or, for short, EMF. So, on a round battery for a flashlight it is written: 1.5 V. What does this mean? Connect two metal balls carrying charges of opposite signs with a conductor. Under the influence of the electric field of these charges, an electric current arises in the conductor ( fig.15.7). But this current will be very short-lived. The charges quickly neutralize each other, the potentials of the balls become the same, and the electric field disappears.
Third party forces. In order for the current to be constant, it is necessary to maintain a constant voltage between the balls. This requires a device current source), which would move the charges from one ball to another in the direction opposite to the direction of the forces acting on these charges from the electric field of the balls. In such a device, in addition to electric forces, the charges must be affected by forces of non-electrostatic origin ( fig.15.8). Only one electric field of charged particles ( Coulomb field) is not capable of maintaining a constant current in the circuit.
Any forces acting on electrically charged particles, with the exception of forces of electrostatic origin (i.e., Coulomb), are called outside forces. The conclusion about the need for external forces to maintain a constant current in the circuit will become even more obvious if we turn to the law of conservation of energy. The electrostatic field is potential. The work of this field when moving charged particles in it along a closed electric circuit is zero. The passage of current through the conductors is accompanied by the release of energy - the conductor heats up. Therefore, there must be some source of energy in the circuit that supplies it to the circuit. In it, in addition to the Coulomb forces, third-party, non-potential forces must necessarily act. The work of these forces along a closed contour must be different from zero. It is in the process of doing work by these forces that charged particles acquire energy inside the current source and then give it to the conductors of the electrical circuit. Third-party forces set in motion charged particles inside all current sources: in generators at power plants, in galvanic cells, batteries, etc. When a circuit is closed, an electric field is created in all conductors of the circuit. Inside the current source, the charges move under the influence of external forces vs. Coulomb forces(electrons from a positively charged electrode to a negative one), and in the external circuit they are set in motion by an electric field (see Fig. fig.15.8). The nature of extraneous forces. The nature of outside forces can be varied. In power plant generators, external forces are forces acting from the magnetic field on electrons in a moving conductor. In a galvanic cell, for example, the Volta cell, chemical forces act. The Volta element consists of zinc and copper electrodes placed in a solution of sulfuric acid. Chemical forces cause the zinc to dissolve in the acid. Positively charged zinc ions pass into the solution, and the zinc electrode itself becomes negatively charged. (Copper dissolves very little in sulfuric acid.) A potential difference appears between the zinc and copper electrodes, which determines the current in a closed electrical circuit. Electromotive force. The action of external forces is characterized by an important physical quantity called electromotive force(abbreviated EMF). The electromotive force of the current source is equal to the ratio of the work of external forces when moving the charge along a closed circuit to the value of this charge:
Electromotive force, like voltage, is expressed in volts. We can also talk about the electromotive force in any part of the circuit. This is the specific work of external forces (the work of moving a unit charge) not in the entire circuit, but only in this area. Electromotive force of a galvanic cell is a value numerically equal to the work of external forces when moving a unit positive charge inside the element from one pole to another. The work of external forces cannot be expressed in terms of the potential difference, since external forces are non-potential and their work depends on the shape of the charge trajectory. So, for example, the work of external forces when moving a charge between the terminals of a current source outside the source itself is equal to zero. Now you know what EMF is. If 1.5 V is written on the battery, then this means that third-party forces (chemical in this case) do 1.5 J of work when moving a charge of 1 C from one pole of the battery to another. Direct current cannot exist in a closed circuit if external forces do not act in it, that is, there is no EMF.
PARALLEL AND SERIES CONNECTION OF CONDUCTORS
Let us include in the electrical circuit as a load (current consumers) two incandescent lamps, each of which has some specific resistance, and each of which can be replaced by a conductor with the same resistance.
SERIAL CONNECTION
Calculation of the parameters of the electrical circuit with a series connection of resistances:
1. the current strength in all series-connected sections of the circuit is the same 2. the voltage in a circuit consisting of several sections connected in series is equal to the sum of the voltages in each section
3. the resistance of a circuit consisting of several series-connected sections is equal to the sum of the resistances of each section
4. the work of an electric current in a circuit consisting of series-connected sections is equal to the sum of the work in individual sections
A \u003d A1 + A2 5. the power of the electric current in a circuit consisting of series-connected sections is equal to the sum of the powers in the individual sections
PARALLEL CONNECTION
Calculation of the parameters of the electrical circuit with a parallel connection of resistances:
1. the current strength in an unbranched section of the circuit is equal to the sum of the current strengths in all parallel connected sections
3. when the resistances are connected in parallel, the values \u200b\u200bthat are inverse to the resistance are added:
(R - conductor resistance, 1/R - electrical conductivity of the conductor)
If only two resistors are connected in parallel in a circuit, then about:
(when connected in parallel, the total resistance of the circuit is less than the smaller of the included resistances)
4. The work of an electric current in a circuit consisting of parallel-connected sections is equal to the sum of the work in individual sections: A=A1+A2 5. The power of the electric current in a circuit consisting of sections connected in parallel is equal to the sum of the powers in the individual sections: P=P1+P2
For two resistances: i.e. the greater the resistance, the less current it has.
The Joule-Lenz law is a physical law that allows you to determine the thermal effect of the current in the circuit, according to this law: , where I is the current in the circuit, R is the resistance, t is the time. This formula was calculated by creating a circuit: a galvanic cell (battery), a resistor and an ammeter. The resistor was dipped into a liquid, into which a thermometer was inserted and the temperature was measured. This is how they deduced their law and imprinted themselves forever in history, but even without their experiments it was possible to deduce the same law:
U=A/q A=U*q=U*I*t=I^2*R*t but despite this honor and praise to these people.
Joule Lenz's law determines the amount of heat released in a section of an electrical circuit with finite resistance when current passes through it. A prerequisite is the fact that there should be no chemical transformations in this section of the chain.
WORK OF ELECTRIC CURRENT
The work of an electric current shows how much work was done by an electric field when moving charges through a conductor.
Knowing two formulas: I \u003d q / t ..... and ..... U \u003d A / q, you can derive a formula for calculating the work of an electric current: The work of an electric current is equal to the product of the current strength and the voltage and the time the current flows in the circuit.
The unit of measure for the work of electric current in the SI system: [ A ] \u003d 1 J \u003d 1A. b. c
LEARN, GO! When calculating the work of an electric current, an off-system multiple unit of electric current work is often used: 1 kWh (kilowatt-hour).
1 kWh = ...........W.s = 3,600,000 J
In each apartment, to account for the electricity consumed, special electricity meters are installed, which show the work of the electric current, completed over a certain period of time when various household electrical appliances are turned on. These meters show the work of electric current (electricity consumption) in "kWh".
You need to learn how to calculate the cost of electricity consumed! We carefully understand the solution of the problem on page 122 of the textbook (paragraph 52)!
ELECTRIC CURRENT POWER
The power of the electric current shows the work of the current done per unit of time and is equal to the ratio of the work done to the time during which this work was done.
(power in mechanics is usually denoted by the letter N, in electrical engineering - by letter R) because A = IUt, then the power of the electric current is equal to:
or
The unit of electric current power in the SI system:
[P] = 1 W (watt) = 1 A. B
Kirchhoff's laws – rules that show how currents and voltages are related in electrical circuits. These rules were formulated by Gustav Kirchhoff in 1845. In the literature, they are often called Kirchhoff's laws, but this is not true, since they are not laws of nature, but were derived from Maxwell's third equation with a constant magnetic field. But still, the first name is more familiar to them, therefore we will call them, as is customary in the literature - Kirchhoff's laws.
Kirchhoff's first law – the sum of the currents converging in the node is equal to zero.
Let's figure it out. A node is a point that connects branches. A branch is a section of a chain between nodes. The figure shows that current i enters the node, and currents i 1 and i 2 leave the node. We compose an expression according to the first Kirchhoff law, given that the currents entering the node have a plus sign, and the currents emanating from the node have a minus sign i-i 1 -i 2 =0. Current i, as it were, spreads into two smaller currents and is equal to the sum of currents i 1 and i 2 i=i 1 +i 2. But if, for example, the current i 2 entered the node, then the current I would be defined as i=i 1 -i 2 . It is important to take into account the signs when compiling an equation.
Kirchhoff's first law is a consequence of the law of conservation of electricity: the charge coming to the node in a certain period of time is equal to the charge leaving the node in the same time interval, i.e. the electrical charge in the node does not accumulate and does not disappear.
Kirchhoff's second law – the algebraic sum of the EMF acting in a closed circuit is equal to the algebraic sum of the voltage drops in this circuit.
Voltage is expressed as the product of current and resistance (according to Ohm's law).
This law also has its own rules for application. First you need to set the direction of the contour bypass with an arrow. Then sum the EMF and voltage, respectively, taking with a plus sign if the value coincides with the bypass direction and minus if it does not. Let's make an equation according to the second Kirchhoff's law, for our scheme. We look at our arrow, E 2 and E 3 coincide with it in direction, which means a plus sign, and E 1 is directed in the opposite direction, which means a minus sign. Now we look at the voltages, the current I 1 coincides in the direction with the arrow, and the currents I 2 and I 3 are directed oppositely. Consequently:
-E 1 +E 2 +E 3 =I 1 R 1 -I 2 R 2 -I 3 R 3
On the basis of Kirchhoff's laws, methods for analyzing sinusoidal alternating current circuits have been compiled. The method of loop currents is a method based on the application of the second Kirchhoff law and the method of nodal potentials based on the application of the first Kirchhoff law.
Conditions for the occurrence of current.
An electric current is a directed movement of charged particles. The quantitative characteristics of the current are its current strength (the ratio of the charge: transferred through the cross section of the conductor per unit time):
and its density, determined by the ratio:
.The unit of current strength is the ampere (1A is the characteristic value of the current consumed by household electric heaters).
The necessary conditions for the existence of current are the presence of free charge carriers, a closed circuit and an EMF source (battery) that supports directional movement.
Electric current can exist in various media: in metals, vacuum, gases, solutions and melts of electrolytes, plasma, semiconductors, tissues of living organisms.
When a current flows, the interaction of charge carriers with the environment almost always occurs, accompanied by the transfer of energy to the latter in the form of heat. The role of the EMF source is precisely to compensate for heat losses in the circuits.
Electric current in metals is due to the movement of relatively free electrons through the crystal lattice. The reasons for the existence of free electrons in conducting crystals can only be explained in the language of quantum mechanics.
Experience shows that the strength of the electric current flowing through the conductor is proportional to the potential difference applied to its ends (Ohm's law). The coefficient of proportionality between current and voltage, which is constant for the selected conductor, is called electrical resistance:
Resistance is measured in ohms (the resistance of the human body is about 1000 ohms). The magnitude of the electrical resistance of conductors slightly increases with increasing temperature. This is due to the fact that when heated, the nodes of the crystal lattice increase chaotic thermal vibrations, which prevents the directed movement of electrons. In many problems, direct allowance for lattice vibrations turns out to be very laborious. To simplify the interaction of electrons with oscillating nodes, it turns out to be convenient to replace them with collisions with gas particles of hypothetical particles - phonons, whose properties are chosen so as to obtain a description as close as possible to reality and can turn out to be very exotic. Objects of this type are very popular in physics and are called quasiparticles. In addition to interactions with vibrations of the crystal lattice, the movement of electrons in a crystal can be hindered by dislocations - violations of the regularity of the lattice. Interactions with dislocations play a decisive role at low temperatures, when thermal vibrations are practically absent.
Some materials at low temperatures completely lose their electrical resistance, passing into a superconducting state. Current in such media can exist without any EMF, since there are no energy losses in collisions of electrons with phonons and dislocations. The creation of materials that retain the superconducting state at relatively high (room) temperatures and low currents is a very important task, the solution of which would make a real revolution in modern energy, because. would allow the transmission of electricity over long distances without heat loss.
Currently, electric current in metals is mainly used to convert electrical energy into heat (heaters, light sources) or mechanical (electric motors). In the latter case, the electric current is used as a source of magnetic fields, the interaction with which other currents cause the appearance of forces.
Electric current in vacuum is strictly speaking impossible due to the absence of free electric charges in it. However, some conductive substances, when heated or irradiated with light, are capable of emitting electrons from their surface (thermal emission and photoemission), which are able to maintain an electric current, moving from the cathode to another (positive) electrode - the anode. When a negative voltage is applied to the anode, the current in the circuit breaks. The described property causes the widespread use of electrovacuum devices in electronic devices for rectifying alternating current. Until relatively recently, electrovacuum devices were widely used as amplifiers of electrical signals. At present, they are almost completely replaced by semiconductor devices.
At first glance, electric current in gases cannot exist due to the absence of free charged particles (electrons in atoms and molecules of gases are firmly “linked” to nuclei by electrostatic forces). However, when transferring an energy of the order of 10 eV to an atom (the energy acquired by a free electron when passing through a potential difference of 10 V), the latter passes into an ionized state (an electron leaves the nucleus for an arbitrarily large distance). In gases at room temperature, there is always a very small amount of ionized atoms that have arisen under the action of cosmic radiation (photoionization). When such a gas is placed in an electric field, charged particles begin to accelerate, transferring the accumulated kinetic energy to neutral atoms and ionizing them. As a result, an avalanche-like process of increasing the number of free electrons and ions develops - an electric discharge occurs. The characteristic glow of the discharge is associated with the release of energy during the recombination of electrons and positive ions. The types of electrical discharges are very diverse and strongly depend on the composition of the gas and external conditions.
Plasma.
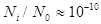
The plasma can also be in thermodynamic equilibrium. For its existence, a very high temperature is required (at which the energy of thermal motion is comparable to the ionization energy). Such temperatures exist on the surface of the Sun, can occur during very powerful electrical discharges (lightning), during nuclear explosions. Such a plasma is called hot.
atmospheric electricity.
Earth is a fairly good conductor of electricity (compared to dry air). At an altitude of about 50 km, ionizing cosmic radiation causes the presence of the ionosphere - a layer of highly ionized gas. Measurements show that there is a huge potential difference between the ionosphere and the Earth's surface (about 5,000,000 V), and the ionosphere has a positive charge relative to the Earth. The presence of a potential difference between the Earth and the “sky” leads to the appearance of a current of very low density (
A/) even in such a poor conductor as air. The total current coming to the surface of the planet is very large (approx. A), and the power it releases is comparable to the power of all built power plants (W). Natural questions arise about the mechanism for maintaining this potential difference and about the reasons why its presence has not yet been used in any way by man.It has now been established that the main mechanism that charges the “sky” relative to the Earth is thunderstorms. Drops of water and ice crystals, moving down to the base of the thundercloud, collect negative charges in the atmosphere and thereby charge the lower part of the thundercloud with negative electricity to potentials many times greater than the potential of the Earth. As a result, a very large electric field arises between the Earth and the cloud, directed in the opposite direction compared to the field that exists in cloudless weather. Near conducting objects protruding from the Earth's surface, this field is further enhanced and is sufficient for gas ionization, which grows according to an avalanche law. The result is a very powerful electrical discharge called lightning. Contrary to popular belief, lightning starts on Earth and hits clouds, not the other way around.
An electric field of 100 V/m, which is characteristic of clear weather, cannot be used or even felt, although at a height equal to the height of a person, in its absence, it creates a potential difference of about 200 V. The reason for this is the low conductivity of the air and, as a result, the low densities of the currents flowing to the Earth's surface. The introduction of a good conductor (a person) into the electrical circuit, shunting a two-meter air column, practically does not change the total resistance of the “heaven-Earth” circuit, the current in which remains unchanged. The voltage drop caused by it on the human body is about U = IR = 0.2 μV, which lies significantly below the sensitivity threshold of our body.
Electric current in living tissues.
The important role of electrical impulses for the life of organisms was assumed more than 200 years ago. It is now known that these impulses are used to ensure the control of the work of organs and the transfer of information between them in the process of life. The role of cables for signal transmission in the most complex "biological computer" is played by nerves, which are based on highly specialized cells - neurons. The main functions of these cells are receiving, processing and amplifying electrical signals. Neurons communicate with each other in a “network” with the help of special elongated outgrowths - axons that act as conductors. Studies of the propagation of electrical signals in axons were carried out jointly by biologists, chemists and physicists in the 30-60s of our century and were one of the first successful examples of fruitful cooperation between representatives of related natural sciences.
As it turned out, the properties of electrical impulses propagating in axons differ significantly from those familiar to electrical engineering: 1) the speed of propagation of impulses along the axon turns out to be several orders of magnitude less than those characteristic of metal ones; 2) after the passage of an electrical impulse, there is a “dead” time during which the propagation of the next impulse is impossible; 3) there is a threshold voltage value (pulses with an amplitude below the threshold do not propagate); 4) with a slow increase in voltage, even to a value exceeding the threshold, the impulse is not transmitted along the axon (“accommodation”).
The listed peculiarities of axon conductivity, which are uncharacteristic for traditional electrical engineering, were explained within the framework of a very specific electrochemical mechanism, the central role in which belongs to a semi-permeable cell membrane for ions, which separates the internal volume of the cell (and its axon) containing an abnormally high concentration of K + ions and low - Na + from an environment filled with saline. As a result of the chaotic thermal motion of particles across the boundary between areas with different concentrations of positive ions, diffusion flows arise (K + - from the cell, Na + - inside it), the speeds of which are regulated by the permeability of the cell membrane and the electrical potential difference on both sides of it. Changes in the permeability of the membrane for each of the ions leads to a change in the number of charged particles crossing the boundary and, consequently, to a change in the electric potential of the axon relative to the external environment. Experiments have shown that the conductivity of a section of the membrane varies depending on the potential difference applied to it. That. the electrical impulse applied to the axon section changes the membrane conductivity for a short time (depending on the properties of the axon), which leads to a redistribution of charges, amplification of the impulse and the formation of its trailing edge. In this case, the axon simultaneously plays the role of a conductor and "amplifying substations - repeaters", which makes it possible to avoid attenuation of signals transmitted in the body over sufficiently long distances.
It is interesting that a very similar problem with the one that was solved by nature, shortly before the discovery of the mechanism of axon conduction, had to be solved in radio engineering when trying to organize a trans-Atlantic cable connection. In order to avoid attenuation and signal distortion in a long line, the cable had to be divided into relatively short links, between which amplifiers were placed. The experience gained by physicists in the creation of long cable communication lines has greatly facilitated the solution of the problem of the mechanism of axon electrical conductivity.